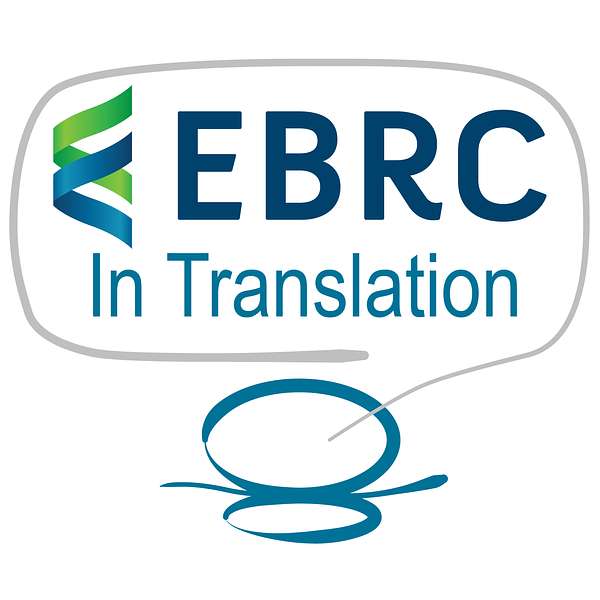
EBRC In Translation
EBRC In Translation
26. Synthetic Biology and Awestronomy w/ Drew Endy
In this episode, we interview Drew Endy, a professor in the Department of Biological Engineering at Stanford University. We talk to Drew about making the engineering of living systems routine, how biology is the perfect technology to interface with joules, bits, and atoms, the concept of synbiophobia-phobia, teaching biological literacy via the “Labrary”, the new discipline of Awestronomy, and so much more.
In this episode, Drew mentions several interesting reads:
The Genesis of a Curriculum on Biological Engineering
The Morals of Measurement
Homo Ludens
For more information about EBRC:
Visit our website at ebrc.org. If you are interested in getting involved with the EBRC Student and Postdoc Association, fill out a membership application for graduate students and postdocs or for undergraduates and join today!
Transcription:
Episode transcripts are the unedited output from Whisper and likely contain errors.
Hello, and welcome back to EBRC in Translation. We're a group of graduate students and postdocs working to bring you conversations with members of the engineering biology community. I'm Andrew Hunt, a postdoc in the Baker Lab at the University of Washington. And I'm Rina Said, a PhD candidate in the Nanai Lab at Tufts University. Today, we are joined by Drew Endy, who is a true pioneer in the field of synthetic biology. Drew is a professor in the Department of Biological Engineering at Stanford University, and he's also known for many things, including his early work on the standardization of biological parts through the BioBricks Foundation. He also helped to start the International Genetically Engineered Machine, or iGEM, competition. He's also founded several companies like Code on Devices and Gen9, and other things as well that we're excited to talk about. So Drew, it's a pleasure to have you on the podcast, and thanks so much for joining us today. Yeah, thanks for inviting me. I'm grateful to be here. So to get us started, could you tell us a bit about your background, maybe starting around your PhD and how you became interested in synthetic biology? I have to go a little bit earlier to make sense of it. I grew up in Pennsylvania, and wasn't totally sure what to do. Probably shouldn't have gone to college right away. I did telemarketing and roofing. Found myself going to college and studying civil engineering, which is short for civilian engineering, and got really into structural design. Reinforced concrete, steel, building stuff, like all that sort of cliche. In high school, I went to public high school, and my experience with biology there was mixed. Sometimes it was memorized the Latin species names of 200 insects, and I got a D that quarter in high school biology, which really messed up my college applications, and I should have gotten an F, and I got a D. And then other times it was camp out at a stream with an oxygen meter and look at the patterns of dissolved oxygen in the creek at 3 a.m. and so on, or do some endocrinology experiments with mice. It's really amazing. When I went to college, I was initially abiotic. I had had this mixed high school experience with biology, and it wasn't until my senior year I had an elective in structural dynamics. What happens when there's an earthquake, or you've got buildings moving, and the professor wasn't able to teach the course. I was looking to substitute with something real, like a hard course. Molecular genetics was being taught at 7.45 in the morning by Vassie Ware, and she's an amazing teacher. I remember she was showing on view graphs three-dimensional structures of molecules, and I was sitting in the back of the small class, the only engineer in this class. It was early 1990s, and I must have had a horrible look on my face. She asked me, like, what's wrong? I said, why do I have to worry about what the protein looks like? Can't you just tell me what it does? I just want to know what it does. I just want to use this stuff. I was seeing for the first time the molecules of life as building blocks, and she was so kind. She didn't say, you fool. There's a relationship between structure and function. She just was sort of like, oh, Drew, you're an engineer, and helped me keep learning. I'm really grateful to Professor Ware. In any case, coming out of undergrad, I had job offers in construction management, because that's what I had done my internships in. Really nice job, pay a lot of money helping to build refineries in the Middle East. Professor Ware and others have lit this bio bug anew, grounded at the molecular level, which I could vibe with. I stayed on and did a master's degree in environmental engineering, coming out of civil engineering. It's within the same department typically, and so the administrative burden was not too difficult to change a little bit. I backfilled biochemistry, genetics, cell biology as much as I could. That taught me that wonderful and well-intentioned engineers at the time weren't really thinking about biology at the level the biologists were, at the molecular to cellular level in this full-on way. I was hunting for PhD programs that might support that. I don't want to say dumb luck, but pretty close to that, stumbled upon the engineering school at Dartmouth College. It's such an interesting place because they don't have departments in the engineering school. All the engineers are in the same school with no department barriers. It's very interdisciplinary. People are excellent. They had taken all their billets for chemical engineers and made a strategic bet on biochemical engineering. They had this little cluster hire of three or four biochemical engineers. John Yin, who had just come back from a post-doc with Manfred Eigen in Germany on virus evolution, was among that group. He was the first engineer I really vibed with who understood this bioengineering evolution. I was very interested. All I could say to myself at the time was, second law of thermodynamics is really interesting. Things tend towards disorder, yet living systems probably create patterns that persist through space-time. What's the intersection between entropy and evolution? I was able to connect with John on that topic and find my first genuine home in what I would see as bioengineering. If you fast forward, I'm returning full circle to my roots as a civil engineer. Biology is nature's ultimate way of growing things, and growing is the best way of building. We're slowly, to my liking, unlocking biology as a general purpose technology, but we're getting there. That's just if you tunnel back from now, but it's a biased random walk with a lot of persistence. Yeah, very fun. Your earlier work in synthetic biology has had a significant impact on the field. Can you tell us about some of your earlier research that you found particularly memorable? Yeah, I started working in what was going to become called systems biology before it was called that, how to create representations of living systems. I was deep enough into the weeds of that that I found the problems. If you go back in time 25 years, I was building computer models of phage infection of whole cells. When I would make predictions using my models, the most interesting predictions were wrong. I could make this change, and the phage will grow faster. Then I'd try and go in the lab to make that change, and I'd make the change, the phage will grow slower. By the way, it's really easy to take an optimized system and make it worse. That's a less impressive prediction, but taking an optimized system and making it perform better is like, oh, wow. Anyway, none of my non-trivial predictions were right. Root cause failure analysis, one of them, there were three reasons that I was failing. One was the physics engine for systems biology is lame, not good enough. It abstracts away physics basically, except for time at the time. The second is we have essential genes of unknown function, and so we don't have complete models, and that's still true. The third thing was the architecture of the system. Evolution is not selecting for natural living systems whose designs are easy for human beings to understand, model, and represent. 1999, this is the pre-represolator era, just to wind back the clock. I'm looking at why my models of natural living systems are failing me, and I start blurting out things like, I'm going to have to rebuild the living world to make it modelable. If I want to have high fidelity models of living systems, I'm going to have to refactor them, I have to rebuild them. I just was walking around Berkeley at the time saying these things, and I was in a crazy enough environment that that was an okay thing to say. When I started broadcasting that, suddenly Elowitz appeared in a different vein, and suddenly Tom Knight appeared, and I remember, just to give you a little bit more context, I remember sitting down at my desk and being like, I have some interesting ideas. Nobody's asking me about them. I'm not getting enough email. Could you imagine saying that? I'm not getting enough email. Maybe somebody's going, what's email? But at the time, email was important, right? I was like, I'm not getting enough email, which was a signal for me. It's like, I need to talk more about what I'm thinking. You start broadcasting these ideas, and they just wait to see who on earth responds. One of the people who responded was TK, or Tom Knight, then at MIT, and Tom blarts out, of course, the code is four billion years old. It's time for a rewrite. I'm going, yes, that's what I mean. That's what I mean. Tom's motivations for synthetic biology are very different. He comes from electronics and wants to use biology bottom up to put dopant atoms in semiconductors with really nice precision, which is still a good idea. But anyway, I just think this idea of refactoring and rebuilding and if you look around wherever you are, if you're listening to this conversation, when engineers work with things, very rarely do we work with things as we find them in nature exactly. We typically take natural materials and remake them at a very fundamental level so that they're easier to work with. That's refining and standardization. So from refactoring, that leads into refinement and standardization to make substrate that's more amenable for engineering purposes. Give you an example. I remember a talk Roger Chen, the late Roger Chen gave in Berkeley around 2000 or so, and everybody was making these GFP fusions to see where proteins would go, eukaryotic cells especially. And everybody was reporting how interesting it was these proteins would have all these pump date structures and they were really sophisticated structures. And Roger said, well, sorry everybody, the wild type GFP is a weak homodimer. And so when you fuse it to your protein of interest, it creates an increased propensity for these collapsed pump date agglomerations to form. And it's an artifact. Because if you have a native protein that's already weak homodimer and you fuse it to another weak homodimer, it crashes together. But then he says, not to worry, if you make the A206K substitution at position 206, you abolish that weak homodimer propensity for GFP. And when we do this, we see two thirds of the reports in the literature go away. But that's a tiny little example of taking a natural thing and refining it to make it a more useful human thing. And so that's the early transition for me into the field and why I got excited about it from my own perspective. And then puts in motion the papers that come out of the lab from 2003 to 2013, all thinking about refinement, standardization, unlocking coordination of labor, culminating probably in the work led by Vivek Mutalik I did in collaboration with Adam Arkin around composition of five prime UTR elements in bacteria to get precision translation initiation. At a point in time where everybody was like, that's impossible. It's like, ah, no, it turns out. But it all comes back to, how are we working with this substrate? And what does it mean to partner with Living Matter and help it to develop as an engineering-friendly substrate? Yeah. Some really fun history in there. Thanks for sharing all that. Can you tell us a little bit about how you're taking that forward now in your lab at the moment? Yeah. I mean, if you go back in time, a lot of the work, 2003 to 2013, is molecular composition at the parts to device or device to pathway or device to system level, all within the context of the cell. And before that, I remember being a PhD student building computer models of natural genomes. I'm like, oh, I want to be designing genomes. And by designing genomes, I didn't mean like minimized microplasma or yeast 2.0. I mean those new creatures. So starting around 2013, to us at least, it felt like we had proven certain core ideas in synthetic biology weren't impossible to make real, around abstraction and composition, the amplifying genetic logic gates paper we did. I regret we didn't point this out in the paper. Every DNA design in that paper worked the first time. There was no design, build, test, learn. It was design, build, work. And that was because in setting up that work, we had properly abstracted analog to digital converters based on DNA flippers that just work reliably. And a lot of people still get those off of AdG, which is wonderful. But so if 10 years ago, we were feeling reasonably good about these core ideas not being impossible, and I'm using those words purposely because they're mostly not made true. They're very little work, frankly, on refinement and standardization and functional abstraction. It's mostly brute force Edisonian empiricism, which I have feelings about. But maybe we get back to that. But for the last decade, it's sort of like, well, what's the next leap? And for us, it really is that leap to the cellular scale. Cells are the fundamental unit of life. And there's no cell on this planet that any homo sapien operationally understands. The simple minimal cells still have of order today 70, 75 genes, each individually essential for viability, and the function is unknown. And so for the last decade, we've been moving up the abstraction stack to the cellular scale and trying to think about and do more than think about get to the beginnings of full operational understanding of engineered cells. And I believe that we're not going to get there for natural cells, or we're not going to get there first for natural cells. We're going to get there first for bottom up constructed cells. So the last cohort or generation of PhD students in the lab, Eric Way, Jonathan Kayes, Anton Jackson Smith, Akshay Maheshwari, in different ways have all been prototyping and pioneering methodology towards building, modeling, imaging, and composing and debugging bottom up constructed synthetic cells. There's a number of teams working on different aspects of this all over the world. Our unique niche is coming at it from the perspective of systems integrator. How do we get everything to function as an integrated whole? You know, since sometimes you think about things from a making and modeling and measuring perspective, maybe I can give some quick examples if you want. Yeah, please. Right. So 20 years ago, incredible researchers pioneered the peer system, protein expression using recombinant elements. So a purified cell-free system, right, as you can well appreciate. Eric Way, a PhD student in electrical engineering here, took inspiration from the Unix operating system, its development in the 70s, a miniature operating system for a mini computer, and asked the question, could pure remake pure? If I add the DNA encoding the molecules comprising pure, do I get my pure back? A lot of people have published on what pure can remake of itself, but Eric actually tested all of pure and found the things pure cannot remake. So like a debugging approach, and he organizes all of this in what we call the periodic table, and there's a preprint on it, you can track it down. But as an example, if you make the ribosome release factors in pure using pure, they don't have all the right modifications to function. And so you'd have to add new componentry, right? So it's like, oh, okay, we have a platform for debugging how to get pure to remake pure. There's another issue, of course, which is pure doesn't have enough oomph, can't make enough peptide bonds. So it needs to be 20 times more active. That can be solved through buffer exchange, I think. Another example is Akshay Mahashwari in partnership with Rosanna Zia, now at the University of Missouri, but then at Stanford, adapting colloidal hydrodynamics physics to represent cellular behaviors, to fill in, you know, for structural biology, we have really good physics going from atoms to molecules. When you get the whole cell systems biology, you abstract away space, right? And colloidal hydrodynamics and the physics underlying that let you represent individual molecules that you might think of as Brownian, but have interesting behaviors. And on those platforms, we've been able to recapitulate and extend a lot of interesting observations about how cells behave as a function of growth rate and volume fraction, meaning the amount of space within the cytoplasm occupied by biomolecules. And so for me, that's looping me back to the 1990s and addressing one of my root concerns with systems biology, that we didn't have a good physics engine. But, you know, I use physics engine like you're playing a video game, like what's the physics engine, right? But now I'm feeling like we've got a rocking physics engine for cell scale systems biology, and Akshay has really pioneered that. Most recently, Anton Jackson Smith has worked with Wachu's group up at the National Lab, Slack, and used the Cryo-EM platforms to get whole images of liposomes encapsulating pure, expressing things needed to build cells. And Anton's motivation is to use label-free tomographic reconstruction as a way of debugging the systems integration problem. So can you tell how things are working just by looking at them and how things are related to other things in space? And it's looking really good, actually. It's just really expensive. But that's okay, because I think we're the first synthetic biology customers for Cryo-EM at this scale. And so now it feels to me, when I take all these projects in combination, we're at the starting line of a next set of work, which is going to require creative PhD students but is also going to require professional engineers. And I estimate that it's, we debate this, it's somewhere between $50 and $100 million of work to build a cell, but to build a cell that's not constrained by any terrestrial lineage. And when I get asked about this in Washington, they're like, well, so confusing. Like, what cell are you building? You're building E. Coli? You're building yeast? And I was like, no, the cell we're building is American. We're building an American cell, and it's more akin to an operating system at this other scale. But we don't have the 100 million bucks right now. So work to be done around telling the story for sure. Yeah, interesting. I think this question taps into like the past and the future. So you mentioned like, the start of like trying to standardize and all this stuff, and then and trying to build and learn in the future, the next leap is to actually build like a minimal cell to modelable cell model. I don't, but I don't like just just to slow down a little bit. It's such an interesting, like, do you want to drive your car across a minimal bridge? No, no, yeah, yeah, I don't. I'm afraid I'm afraid of heights. I don't, right? I want to drive my car across a modelable bridge. Minimal and modelable don't, aren't necessarily the same thing. Yeah. So as someone who has witnessed all these like things, what are the biggest successes? Have you seen this technology so far? I remember you mentioned like GFP, all these kinds of, but what are the biggest success from your lens? And also, did the synthetic biology went as you expected, like the progression, like comparing the past and the future? How do you see it right now? How do you think? Is it heading in the right direction? It's a good question. Would you consider a synthetic RNA vaccine made via DNA synthesis a success of synthetic biology? Why or why not? I think it's, yeah, it is a success. Yeah, I'd also agree. I think there's lots of things that were drawn from synthetic biology, and I think the boundaries of what we call synthetic biology are a little bit fuzzy, it's hard to tell. Yeah, but I just want to pause and come back to that example. Is a synthetic RNA vaccine a success in part or in whole of synthetic biology? And why are many people not comfortable going, yep, right? One reason is publicly in United States, at least people are discomforted by the apparently rapid innovation of the vaccine, right? But of course, people were working on that heroically for decades. It's so interesting to me, you know, Nubar Afeyan, who's somebody you should chat with, right? He was the founding chair of Codon Devices, and he and the investors are out $100 billion on that, right? But he's also the chair of Mode RNA, and Moderna has done pretty well, you know, building synthetic RNA vaccines on the back of DNA synthesis. I don't want to take any credit for any of that, but it's just interesting to see some of these loopings and relationships. I am hopelessly biased, but I find the work that Prashant did in the Smoky Lab around yeast-based biosynthesis of scopolamine to be ridiculously awesome. I remember being on his thesis committee, and I wish we had recorded that Zoom for his defense. One of the people in the audience was a director at the Max Plan, and at the end, the person just basically got up and started cheering and declared Prashant his hero for doing things. But if you track down this paper around scopolamine biosynthesis, which is of order a 30-enzyme pathway, there's only two authors on the paper. One is Prashant, and the other is Christina, and I know for a fact Christina wasn't in the lab doing the work, and so somehow one person, like one PhD student here, was sufficient to realize a 30-enzyme pathway in yeast to make a sophisticated plant-natural product, and that's because when you look in the methods, the biosynthetic modules were properly abstracted or abstracted well enough that he could reuse them and benefit from coordination of labor, drawing on his past work, and it's like hmm. So I find that somewhat incredible. Not surprising, but wondrously incredible. Knock on wood, it's interesting to see the Anthea team. Again, I'm hopelessly biased and conflicted, but they're reporting industrial-scale fermentation with commercial titers for these things, and everybody thinks it's so weird. Everybody thinks it's impossible until somebody does it, and then it's suddenly normal, and so to be on that precipice, I also find it remarkable what Mycoworks is doing with synthesis is a different scale around the leather surrogates and their $100 million facility down in Union County, South Carolina, and hopefully is a successful translation to commercial-scale production for a leather surrogate platform. Yeah, but come back to the iGen. What do we make of Ingrid Swanson and her team out of Seattle, Washington that was working on celiac disease through an enzyme re-engineered to work at different PHs that goes right from iGen into a seed investment from the Japanese pharmaceutical house that ends up acquiring them? We use that example with the undergrads here at Stanford because they're so smitten with computer science and this idea that within less than a decade you could go from an undergraduate or student-led project to a multi-hundred million dollar acquisition. They know how to do that in computer science, but they don't know how to do that in bioengineering, and Ingrid is showing them that. So anyway, I would say to your other part of your question, you know, it was 20 years ago, two days ago, so we're talking on a Tuesday. So this past Sunday, 20 years ago, I was briefing the DARPA director on synthetic biology. I had led a 18-month study with 50 amazing colleagues on that, and that briefing, you know, normally you go in and do one of these briefings, and if it goes well you'll get a hundred million dollar program, you get to go run, right? But this briefing was the opposite of that. It was like the Hindenburg coming into New Jersey bursting into conflagration and crashing and horribly, you know, causing harm. That's what this briefing was like. I didn't get halfway through the slide deck. I didn't understand how bad the Amerithrax, anthrax attacks politics had turned biotechnology into a security issue. The only thing that the person receiving the briefing could understand is I was increasing political liability and potentially biosecurity risks, and so there was definitely no new program started, and even worse, there was a program at the time on advanced DNA synthesis with the goal of making a 10 kilobase fragment of DNA in 24 hours, including using novel chemistries like a peroxide-based chemistry that would be more environmentally friendly, and a side effect of my briefing was to contribute to getting that program cancelled. So people who had money to do that research had their money pulled back, you know, like they've got negative money, which is why it was so important when Nubar came in and helped organize the capital to underwrite codon, because the government certainly wasn't going to pay for it, right? It's crazy to think about, and so, you know, I used to wonder I'm always a student of the history of emerging technologies, like the telegraph, you know, like digital abstraction appears in Morse code in the early 19th century, dot and dash, dot and dash, it's a digital abstraction, and that happens before the ohm gets developed as a unit of resistivity for quantifying the properties of wire. Why did it take so long to go from the digital abstraction of the dot dash to the ohm? And I was like, how come humans are so slow at building out the engineering foundations of new technology? Why was it a century until Shannon shows up with relays and logic, right? It's like, why is it so slow? Well, now I know, right? At least the last 20 years, give me a hint. It's mostly because people tend to be overdriven by the immediacy of need and application and have a very hard time sustaining investments for fundamental engineering research. It really only happens when there's no other choice. At least that's what history says. And it's kind of a bummer, right? You know, it's like, hey, the last one of the last conversations I had at MIT before I left was like, hey, I hear what you're working on, synthetic biology is really important. What disease are you studying? You know, it's just like face pop, like shame on me. I didn't know how to explain at the time. It's like, why would I pick one? Let's like make it so that so many people can work on diseases. You don't even have to ask me that question because they're all resolved, right? So- I really love the concept of fundamental engineering. I think it's not talked about enough. Do you know? Check this out. I double dog dare you or anybody to track down a used copy of this book, The Morals of Measurement. It's a historical analysis of what was going on around telegraph engineering and like, how do you get to the home? So, you know, like when your telegraph between Cambridge and Oxford fails and you have no standards around manufacturing or measurement of wires, where do you send the repair crew? And you don't know. And so what you do empirically is you apply Newton's bisection method. You go halfway between the two towns. You take another measurement to determine is it towards Cambridge or back towards Oxford, right? And then you keep bisecting the remaining distance until you isolate a section of wire that's small enough you can afford to splice in a patch. Ta-da! Right? That's telegraph maintenance. And that works well enough until you try and do things like put a telegraph across the Atlantic Ocean. And when you go to apply Newton's bisection method to the ocean, you go bankrupt and you create a political conflagration with your investors that forces the egos at the Royal Academies of this and that to get out of the way and like just unilaterally declare these hunks of metal to be the home, right? But it's like, why is it so slow? That's why it's so slow. It's crazy. Yeah. Really interesting. I think ultimately I'm just, I find myself really interested in these more fundamental engineering questions and like improving the ways that we build things. And yeah, I would love to see more funding in this area. I am so, I need to say something else. There are a lot of academics who are very well established, who are very good at complaining that there's been little progress in the engineering fundamentals of synthetic biology. And they're right in complaining, but they need to look in the mirror a little bit more and acknowledge that they aren't doing any of the work. Yeah. Fair enough. So maybe, maybe this is a great time for our next question, which is sort of, what do you see as like the next biggest challenges and opportunities in synthetic biology in the next decade or so? It's easier for me to think about myself than a field. And so let me do that. I think the physics of flourishing are very, very good. I think it's for the first time in the history of Homo sapiens within reach to equip and enable 10 billion Homo sapiens to flourish in partnership with the rest of life on earth without trashing the place and to accomplish that goal by or before the year 2050 within a human generation. And biology is a critical player in all of this because biology operates at the intersection of jewels, bits and atoms on a planetary scale with enough energy going through it to get the job done. And so I'm working backwards from 2050. And what do we need to do this quest on the edge of possibility between now and then to get to flourishing by or before 2050? And it feels to me like it's just barely not too late. But if we were having this conversation in 2030 and certain things weren't accomplished in the 2020s, it probably would be too late. So we need to routinize the engineering of living systems, i.e. make routine. The idea isn't design, build, test, learn. That mantra needs to get kicked to the curb. The correct statement is design, build, work. You don't keep saying it over and over again. It's just like you design something, you build it, and it works. And for that to be true, between designing and building has to be testing through modeling and re-presentation. When I design a building, it's not a research project. It does have uncertainty around the Gantt chart and where everything will arrive, but it's not a scientific research project. And so how do we get to design, build, work for living systems? And especially at the cellular scale. And my view is we've got to learn how to build and operate cells from the bottom up. And I think we're within striking distance of that. It's of order $100 million attempt. And maybe it fails, but if it fails, we know we'll discover why it fails. We'll just keep going. So I actually would expect that by before the end of this decade, we have routinized the construction of cells from purified molecules, at which point we can get to an operating system for living systems, at which point you could imagine a bionet. So let me pause there and come at this and we'll intersect with bionet again. I do not think most people are conceptualizing a biotechnology correctly. We think of it through the practice and metaphor of industrialization, concentrated energy, concentrated factories, concentrated capital, concentrated power. And then we feed that new supply chain into contemporary industrialized society. But the leaves on the tree await no presidential executive order to start growing. They await no Minnesota bio-maid. They await no factory line to start to go. The leaves on the tree just grow because the energy arrives there and the molecules are there and arrive there. And the biology grows with the energy and materials that are local. Biology teaches us all atoms are local. And if we unlock it right, we've got the ultimate deglobalized, decentralized manufacturing platform. So that's interesting. What if we're not thinking about biotechnology correctly? Another thing is we tend to think of biology as a technology only in a context where it goes in abiotic things. So like a yeast in a fermenter or an RNA vaccine in a chemical lipid nanoparticle that goes in a plastic steel syringe. That's why the vaccine is hard to manufacture. It's not biological completely. It's mostly not biological. Like anything that's not biological doesn't grow exponentially. Why aren't we talking about Roy Curtis III's work with Salmonella engineered to vaccinate against flu? Ten years ago he pioneered that. It's very heartening to see Michael Fischbach's work with engineered staph epidermidis to present antigens for training the immune system against melanoma. So biology as holistic technology that's embedded within living systems feels incredibly important. So zooming out and coming back in, if we routinize the engineering of biology at a cellular scale and we learn how to embrace and make safe and responsible and reliable wholly biological biotechnologies, then we can combine that through DNA sequencing and synthesis with the superpower of the internet. We can combine the superpower biology with the superpower of the internet. So by that I mean the superpower biology is all atoms are local, grow with the stuff that's where the stuff is, and the superpower of the internet is to move information around space time, decouple it from one place, move it to another place. And what that means is instead of like take your Apple computer designed in California made in China, you could get to a future where the DNA is designed anywhere in DNA design studios, then it's distributed and you get download and grow everywhere or anywhere as well. Design anywhere, grow anywhere. And that's going to be the bio net. And so my view is if I come back to this 2050 mindset, this decade, and we have to behave very differently than the last 20 years, this decade we have to develop the essential capacities to enable biology to contribute to planetary scale flourishing. And I'm going to call this like unlock the bio net. And then in the 2030s we need to see these abilities deployed where they need to be responsibly. And in the 2040s it's let's get the job done, enable and equip people to get to flourishing. And it won't be so bookishly staged, like 2020s develop, 2030s deploy, 2040s do, there'll be overlap. But that's what it feels like the opportunity is. One of the big challenges here is we're not very good at talking about where we're going. We're not very good about sharing dreams. It turns out that dreams are a type of technology too. They're a technology we use to organize ourselves and others. And when people pop up into the public sphere and start talking about dreams, they usually take the cheap way out and go with the nightmare. Vice President Gore made a structural error when he said climate change is an inconvenient truth, as opposed to an opportunity. It's easier to market a nightmare, but the hidden cost of the nightmare is you won't get sufficient collective action until enough people believe the nightmare is real. So climate change by that framing has made climate crisis and climate emergency inevitable. Whereas Martin Luther King and Kennedy with the Apollo project give us examples of positive aspirational dreams in advance of a reality that can allow a movement and co-laboring towards that better future. It's much harder to do, it's truly risky to say the least, but I think that's a challenge we've got. Can we articulate flourishing or some collective positive vision in a context where everybody's going to be playing the nightmare card collectively to maintain regimes of scarcity? Yeah, I'm much in there to unpack. I really love the vision. Yeah, I have a follow-up question, like from my humble experience, like having a minor in biotechnology, masters in biotech, and people were like, in my studies, you need to have this lab-to-market transition, because having this in a large scale to be a biotechnology is something else, and I had to take entrepreneurship classes and stuff to be able to have all the parts in. But then you introduce this interesting concept of why we don't learn from the biology of self-propagating. Why can't we just combine the lab skills, like proof of concept and stuff, to the large scale thing? So my question is, what is, if you provide a new definition for biotech then, what would it be? Is it bionet? I don't know if it's a new definition for biotechnology per se, but I can give you a historical example that may lead to something for our consideration. So 50 years ago, there was a revolution in computing across the creek from where I am today in Menlo Park, California. The computer became personal. The revolution in the personal computer is not in the science of the transistor at all. It's hardly at all in electronics even, the low-level electronics. It was built from mostly off-the-shelf stuff. The revolution in the personal computer is in the word personal. It changed who could compute where for what purpose. And it complemented the industrial computer. And I want to emphasize that word. It complemented the industrial computer. And so there's a trap in what I'm saying for myself and anyone, which is to imagine that a different type of biotechnology might be competing with industrial biotechnology. But what would it mean for biotechnology to become personal? And personal could be literally for you, or maybe it's for your family, or your village, or your house district, depending on where you are. And so what's needed for biotechnology to become personal is maybe a friendlier and more useful way for how I like to think about it. Changing the terms and conditions of biotechnology. Who can biotech where for what purpose? I think the bionet, in a way, is inevitable. It already exists. I can give you my easiest and sometimes most alarming example. SARS-CoV-2 got to Switzerland first because a researcher in China uploaded the genome sequence and a researcher in the Swiss capital downloaded it and rebuilt the DNA and transfected cells and recovered live virus in a lab, safely, about two weeks before the pandemic came in over the Italian border. So that's an early bionet packet transaction. But there's much more interesting ones to imagine in the future. So my next question is another type of building. So you were involved in launching some of the first undergrad majors in bioengineering, both at MIT and Stanford. So what lessons did you learn helping in the vision for starting these programs? A lot of people led the construction of that, like Linda Griffith and Doug Laufenberger at MIT, Scott Delp and other colleagues here at Stanford. The lessons that I appreciate include not starting a new engineering major gratuitously. So there's some pre-context which is important. In the 1980s, the Whitaker Foundation correctly declared biomedical engineering was going to become really important and the United States didn't have enough biomedical engineering departments. They wanted to triple the number and probably for a charity, they bravely declared as a board, they're going to decapitalize the endowment to write checks to help universities start these departments. And they spent a billion dollars successfully tripling the number of biomedical departments. Two places said no, thank you, MIT and Stanford, maybe others, but those are the two I know about. And the reason was at the time, neither school could articulate how biology was ready to become a new and distinctive engineering discipline. So one thing is to not start something new gratuitously, but to understand when and why it might become something substantial. And in the case of both schools, among other things, it's synthetic biology plus an appreciation that the physics of living matter are different than the physics of other materials that engineers are adept with. Self-mixing Brownian motion is very different than semiconductors. So gratitude and appreciation for that. I think another thing that was incredible was to listen to the matriculating teenagers. Students are from the future because they're imagining the world when they're adults and they're appearing at an earlier time. So they actually are from the future. They're these strange type of time travelers. And so in the faculty meetings at MIT at the time, 2002, 2003, such as it was before the department, I remember conversations that were wonderful where at the chalkboard, somebody would draw a circle and label it biology. And then somebody would draw another circle with a little bit of overlap and call it engineering. And we'd all gaze longingly at the intersection and go, biological engineering. Yeah. And that's pretty good for faculty. But the teenagers matriculating, they're like, well, there's electrical engineering. I could learn how to design and build a computer. Biological engineering is going to teach me how to design, build a life form or debug natural programs and cure diseases and save the environment. That's what we're doing. Of course, that's what biological engineers. And then the other thing is, if you talk to the students who've been around MIT for a while, they could point you to things that the faculty don't know about. Did you know there's a historical document written in the 1930s with the title On the Genesis of a Curriculum in Biological Engineering at MIT by Carl Compton, then the president? And it outlines a fully baked five-year curriculum where you get a double degree in biophysics and biological engineering in the 1930s. The students knew about that and they pointed us to it. It's like, oh, okay. So one thing is be grateful for not rushing it. The next is listen to the people who are the time travelers, the students, and then just be genuine about it. And then one more thing is, by being genuine about it, recognize that you're not going to get it right right away. It's going to be an iterative process and you can co-develop what the field is becoming through teaching and research both and by being honest with the students about what can't be done yet but co-laboring towards unlocking that. Yeah. I think those are the big lessons. I like how he described the students to be the type of time travelers. So yeah, maybe also related to education, you've been deeply involved in an iGEM. I'm wondering if you have any other things you'd like to share that you feel like you learned there helping to start iGEM with others? Well, remember that the U.S. government 20 years ago was not particularly excited about supporting synthetic biology. And so that's, what do you do? And the lesson becomes, work with the people who want to work with you. And so the people who wanted to work with us were the students. And so MIT has this amazingly wonderful thing called the Interactivities Period, IAP, where January at campus, it's not fall semester or spring semester, it's IAP. And anybody can teach a course on any topic. And so we just started offering the MIT Synthetic Biology Lab modeled after Lynn Conway's VLSI Electronics Labs in the 1970s that she also taught at MIT. And the lesson is, oftentimes when you're trying to do something new, you can say, oh, this famous established person is the person who needs to help me, right? Because if only they would help me, I could do this thing that I need to do that's the new thing, right? It's almost always a mistake. Because the famous established person is established, which means they've already done the new thing they're going to do, and they're really busy. And even if they want to help you, it's going to be hard for them to help you very much, right? And it's very easy to fall in this trap. Like, I'm going to do this new important thing, I need this famous established person or people to help me. It's almost always a pit of infinite despair when you go down that path. Instead, the lesson is, work with the people who want to work with you. So 20 years ago, the government didn't want to work with us, but the students did. So let's just work with the students and figure it out. So that's important. You know, more recently, we've been working with the National Education Equity Lab. I teach Introduction to Bioengineering at Stanford to about 150-200 students with Jen Brophy. It's only 150-200 students. That's not going to get us to flourishing fast enough, right? And so the National Education Equity Lab is a platform that allows high school students at low-income high schools to take college courses for college credit. And so we piloted Intro to Bio at Stanford to 10 high schools across the country last year. That didn't totally fail. It was pretty good. There's a lot of work, but like, we'll figure it out. I think a lot about citizenship and democracy in relation to flourishing. And so when you start to go down that path in a political theoretic sense, it becomes apparent that for people to be citizens within a democracy at a low level, they have to have optionality with respect to accessing essential capacities. If there's something I need to survive and you're the only person I can get it from, you can oppress me. But optionality, having options, gets around that. We're familiar with this in the marketplace around monopolies, but it's deeper than that. So we now think of literacy, reading and writing as a basic human right through the United Nations. That wasn't true very long ago. We think of computer science for all, reading and writing computer code, as an important skill to give everybody the option of learning. What about reading and writing DNA? It's more important. Everybody should have the option of learning to read and write DNA. Oh, there's all sorts of questions that come along with that one. But nevertheless, if you want to have a democracy, we've got to puzzle it out. So something you had mentioned that you were excited about talking about is that you've previously drawn a distinction between different terms to describe our field. So things like synthetic biology versus engineering biology versus biotechnology. So could you talk a little bit about how you see these as distinct and why terminology is important? Yeah. Genetic engineering, synthetic biology, engineering biology, shiny happy biology. What's in a name, right? I mean, you're at the EBRC podcast, right? Engineering Biology Research Consortium. Why is it called that? Where do these names come from? Genetic engineering is the oldest of the set, right? Going back 50 years or so. And so it occupied a space in the etymological landscape. It had an entry in the dictionary already, but it wasn't inclusive of everything that an engineer could imagine. And metabolic engineering is another example, right? So if it's genetic engineering, what are the units for gene expression? Where's my OM? Why is this all research still? It's not like in structural engineering, I go to the back of the book, I get the lookup tables for rebar, and it's going to work when I put that in concrete. None of that's in place. So genetic engineering, which is a great name, had a whole bunch of engineering missing from it. And metabolic engineering, which is another great name, had a whole bunch of engineering missing from it. Not that they didn't have incredible engineering in it and still does, right? And one of the things I have to be really cautious about is in opening in this way, that's not a dig at the incredible history or the incredible accomplishments of genetic engineering or metabolic engineering. It's just, that's not the whole show, right? Some things are missing still. Synthetic biology for me as a name shows up because we were struggling to create space in conversations and thus in laboratories to do new types of work. And at the time, I was a research colleague with Rob Carlson at the Molecular Sciences Institute in Berkeley. And one of the words we were using was, well, we just want to work with biology in accordance with our intentions. And so we started using the words intentional biology. We even created this really lame website, intentionalbiology.org. And what we quickly learned was that was offensive to the biologists because by implication, if we were doing intentional biology, they were doing unintentional biology and they didn't like that. And so Carlos Bustamante from Cal was visiting one day and he was listening to us and we were explaining this immune reaction we had. And he said, it sounds like what you're talking about reminds me of synthetic chemistry. What about synthetic biology? We're like, yep. And mostly it was an empty spot in the dictionary, which is what we needed. We needed a place to do new work. And sometimes you just need to use a new name. Now, the word synthetic biology turned out to be problematic apparently. Maybe it's because the first syllable of the word synthetic is sin. I don't know. There's definitely something that people have written about. It was like, oh, synthetic biology is a polarizing topic with the public. Really? I don't think that's true because I don't think the public knows very much about it all. I think it's a polarizing topic with policymakers who are afraid of the public. I think there's such a thing called synthetic biology phobia. The fear of the fear of synthetic biology. And people have written on that. It's very important writing. But so from the very beginning of using these terms, we immediately confronted maybe we should rename the field. And we thought about that a lot and still do, which is why I remember the term shiny, happy biology. How about we just call it that? Shiny, happy biology. It's going to be great. There's nothing to worry about. And we thought that was a little bit or more than a little bit irresponsible because honestly, biology kills people by a lot. All this conversation about AI being risky, SB is way riskier. And even the AI people will agree to that if you're in a room together. They just like the attention they get because they scare people as well as exciting people. But anyway, so we were part of the Synthetic Biology Engineering Research Center. And one day an email came out that said unilaterally, the government has told us that the name of our field going forward will be engineering biology. And I lived through that transition. And the consequences of that transition included like 40% of the community said, no, thank you, and went away. Why did that happen? Well, believe me, I'm an engineer, true and true. I got no problem with engineering biology. I wrote a paper called Foundations for Engineering Biology. Love it. But I lived through that moment of unilateral renaming, or at least attempted unilateral renaming. And I've debugged where that attempt came from and why under the Obama administration. And I don't want to get into all of that here. But the consequence of that renaming really destroyed a community that was operating at the intersection of engineering and science. So let me give you something to think about. Imagine you were heading over to the chemistry department and you were talking with the faculty and students and postdocs in chemistry, and you unilaterally told all the synthetic chemists, okay, from now on, you're engineering chemists. You're doing engineering chemistry. What would happen? What would you expect would happen? Well, maybe some people would be, if you got money, fine, right? Which some people would do. But other people would be like, no, thank you. And then other people would be really upset and worse, right? They wouldn't talk to you anymore. Synthesis, as I fast forward to today, I used to think in synthetic biology, biology was the more important word. But now I actually think synthesis and synthetic is the more important word. It's such an interesting word. When you go back and look at the root, the etymology of the word, it's about a putting together. It's about composition. Synthesis as a theme is very human. So is engineering, but synthesis is broader and more inclusive. And it's inclusive of science, it's inclusive of culture, it's inclusive of the arts in a musical synthesizer, right? In a way that engineering is not. And so again, I got nothing against engineering biology. I'm a bioengineer, or I would be if we understood biology enough to be real engineers, not yet, right? Well, we're getting there. But it's just an exclusion, a very exclusive word, very excluding word. And so that makes me a little bit sad. And I like synthesis. But it's the problem, the problem, and it's all good, except when people actually believe the government and the bureaucrats who say, oh, we're now moving everything that used to be under this label into the new label, and we really did that. No, you didn't. And it puts the people who are in charge of the newly named thing in an unfair position of feeling like they have to represent this other thing, which they're not, right? And so I worry when that plays out. And because it's not right, and it misses things, and it's exclusionary. Anyway, from the synthesis perspective, I guess we're back in business because of a pandemic and things being made real, and it's becoming more and more important. And other countries who aren't so caught up in etymological juggling conundrums are just moving out. So here we are. But names, names do matter sometimes because they create space for the new thing. Other times, because they let people work together in new ways, or represent and reflect different tribes and cultures. That's why we have words. They matter. Yeah, certainly. Thank you for sharing all that. And I really do want to remind everybody of synthetic biology phobia phobia. The fear of the fear of synthetic biology is a bigger deal than the fear of synthetic biology. I think that's a very good entry point to my next question. So we think that synthetic biology is a polarizing topic with the public. And why do you think that? And how do we get the public to feel like it's a shiny, happy biology? Well, first of all, it's not shiny, happy. It's quite tricky. It's dangerous. It's problematic. You've heard of bioterrorism? Scary, right? You've heard of, have you heard of bio error? Like lab leaks, lab mistakes, bio error. There's bioterror, bioterror, like bad people causing harm, bio error, like researchers making a mistake in a lab. Where did that pandemic come from? Oh boy. All right. And then there's bioscare. Have you heard of bioscare? Okay. So my wife's afraid of spiders. I'm afraid of falling from heights. I'm afraid of falling from heights. I don't know why she's afraid of spiders, but I guarantee you she is. I don't know why I'm afraid of falling from heights, but I definitely am. And what's interesting is right now you're both nodding. You're not laughing at me for saying this. But if I went online and started to express my fear of biotechnology, what would I encounter? I would encounter 170 or more Nobel laureates yelling at me, saying that I am causing harm, making a correct utilitarian argument that biotechnology mitigates suffering. And they're right.