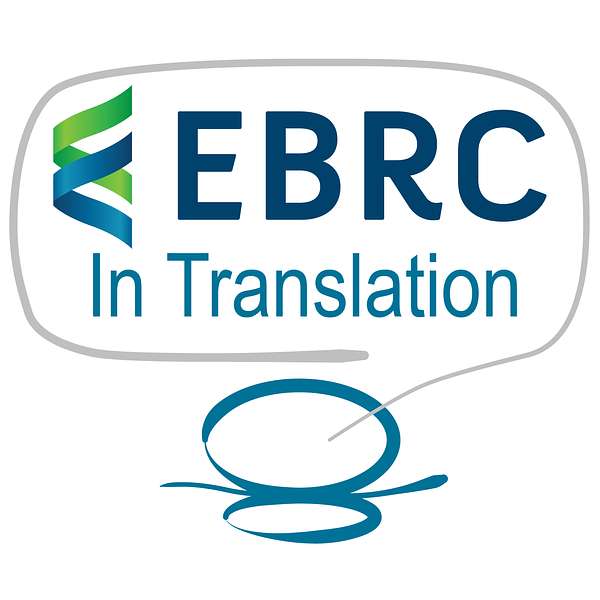
EBRC In Translation
EBRC In Translation
12. Carbon Dioxide Fixation and Carb Consumption w/ Tobias Erb
In this episode, we interview Dr. Tobias Erb, a member of the Max Planck Society and Director of the Max Planck Institute for terrestrial Microbiology in Marburg, Germany. We talk to Tobias about building synthetic carbon dioxide fixation pathways, similarities and differences between science in the US and Europe, and the superiority of German bread and beer.
For more information about EBRC:
Visit our website at ebrc.org. If you are interested in getting involved with the EBRC Student and Postdoc Association, fill out a membership application for graduate students and postdocs or for undergraduates and join today!
Transcription:
Episode transcripts are the unedited output from Whisper and likely contain errors.
Hello and welcome back to EBRC and Translation. We're a group of graduate students and postdocs working to bring you conversations with members of the engineering biology community. I'm Kevin Reed, a graduate student in the Alper Lab at UT Austin. And I'm Katherine Brink, a postdoc and Megan Palmer's group at Stanford. Today we're joined by Tobias Erb, Director at the Max Planck Institute for Terrestrial Microbiology. We're very excited to have you here, Toby. Thank you for joining us. Thank you for inviting me. I'm excited too. It's an experiment for me. I like experiments. Great. To get us started off, how did you become interested in engineering biology and what was your path to becoming a professor in this field? Wow. All right. So I think my interest comes from me being trained in chemistry and biology. And so if you're trained as a chemist, you kind of are really interested in the fundamental understanding of what is matter. And, you know, you take this understanding to then create new molecules, you create new materials. And I think the same principle I've applied to biology, you know, understanding how does life work. So what are the limits of life, living systems, what are the principles, how they operate. And then you use synthetic biology to engineer now new to nature functionalities, pathways, enzymes. And I think it's just a matter of being trained as a chemist going to the synthetic direction. I also think that overall what excites me most is this fundamental biology that they're going from the analytical descriptive way into the synthetic constructive direction. And this is super exciting for me because you explore new fields, new biology. And I think this is at the end of the day convinced me to go into engineering biology. Great. And a quick follow up. What was your path to becoming a professor? Did you go through a few different postdocs? Well, I don't know how I ended up where I am right now. I think I've always been a scientist. I always picked the coolest projects I could imagine, you know, and I tried my best to really advance as much as possible and did my PhD in Germany, had a stint in the US during that time, then decided to go for a short postdoc and returned back to Europe, starting in Switzerland, my own research group with two or three PhD students, and then moving on up the ladder. And here I am now. I think it's just passion, curiosity, and just try as hard as you can. That's really great. We've seen that a lot of your recent work has focused on carbon fixation. So could you tell us a little bit about why carbon fixation is important, and also why biology is a good solution to this problem? Right. So how can carbon fixation not be important? If I start off, if you think about it's basically the fundamental principle of living things, right? It's the conversion of inorganic carbon into living organic matter. So that's what drives life on this planet. And it has basically made the planet what it is. And so just to understand, how does this happen? So what are the enzymes, the pathways? How does carbon dioxide get fixed and then converted into amino acids, nucleic acids, the sugars? That's exciting for me, first of all. Second of all, of course, it matters very much in these times, if we think about planetary scales, if we think about the carbon cycle, how we human beings influence it, right? And how we are challenged by the effects of climate change. And again, like understanding the fundamental principles, how a simple enzyme can capture and filter atmospheric CO2, grab it, and then convert it is fundamentally important if you want to understand on a global scale. And last but not least, I think, if you ask me about biology, what role biology plays, I would say it plays probably the biggest role, because it is fixing CO2 on a gigaton scale, 100 gigatons of carbon, 400 gigatons of CO2 per year. And this is something what we as human beings cannot do. And so learning from nature, how nature can do this in a sustainable way is probably where I got excited about. I think we can learn a lot from biology in all our efforts to try to develop sustainable ways of fixing CO2 in the future. I think also that in principle, we typically see CO2 as a dead end as a greenhouse gas as a challenge. But of course, it is probably the simplest carbon source you can harness, right? And nature actually turns this around and takes it as a building block of life. I think this is something we still need to achieve as a society and as scientists and as mankind. So maybe following up on that a little bit, nature is really great at carbon fixation and taking carbon dioxide and turning it into useful things. Why should we do this synthetically? Is nature not already the best at doing carbon fixation? That's a good question. Nature is great. But you could ask the same question to chemists. Why do we have plastics? Or why do we have other materials, right? Why don't we simply carve everything from wood? So you know, it's a good solution, biological CO2 fixation, but it's not necessarily made for us human beings. I give you two examples. Example one is, if you think about the outcome of biological CO2 fixation, this is biomass, right? It's a very complex mixture. And typically we human beings and industry would like to have a defined product, right? It's one compound you want to make from CO2. And you want to use that. So now if you have biomass to extract this one compound, this is pretty, pretty complicated. So again, I think we can do better if we channel into one product. And the second point is, let's say the carbon fixation rate is great in biology. I just talked about gigatons of CO2, but it's still not there where it could be, right? So it is a process that evolved for billions of years. It's a optimum, but maybe not the optimum. And I would say and argue that we can make CO2 fixation better and faster and more efficient. And this is exactly where we come into play. And again, if you think about global scales, we probably have 100 watt per square meters in Germany and Europe of sunlight, and less than 1% gets converted into actual energy rich compounds. And I think we lose 99% of the energy. And if we tune a little bit in the efficiency of these pathways, we might become much, much, much better and much more efficient. And this is exactly where it could also compensate anthropogenic CO2 emissions at the end of the day. So could you talk about how your lab's work fits into this bigger process of being able to make these pathways more efficient, and being able to capture some of that carbon dioxide that's already in the atmosphere or using sunlight to be able to drive these processes? So in principle, what we ask, the first and foremost, the most important question is what limits natural CO2 fixation? And I could ask evolution at time, billions of years to evolve CO2 fixation. This is correct. But of course, evolution takes only what it knows, right? And so with the billions of years, there's not a leap invention. Typically, it's the first process that becomes better and better and better. But nature rarely invents a complete new solution. And so it very much depends what the first CO2 fixation pathway looked like, how easy could it be established, right? And how easily did it interact with, let's say, photosynthetic apparatus, right? And so nature had only limited amount of time, limited amount of resources to check out a couple of pathways. And I think what we try to do is now to think outside of the box, don't look at what has nature evolved, but look at what nature has not evolved, right? And try to combine enzymes in a better way, more specific enzymes, faster enzymes, enzymes that need less ATP, that need less energy pH. And then you actually have a thermodynamic optimum, you need less energy to fix the same amount of CO2. And if you're also on the kinetic side, and you can fix more CO2 per time, you become better. And this is now our aspiration. We first think about how optimal pathway would look like. We then would go ahead and try to find individual enzymes to build our design. We try to optimize it, for instance, using machine learning, using lab automation. They would like to implement this optimized pathway in the background of a living cell and use natural evolution to further push it. And in principle, it is just exploring the things that nature has not explored during evolution. I think there is an optimum we can still get there. That's great. Yeah, I think one of the awesome things about that, and from watching one of your lectures, is that point where you've taken enzymes from like many different organisms and sort of combined it into that synthetic catch cycle that you guys have done. Could you speak more about that? Of course, I'm happy to do so. So I talked about the limitations. And it's interesting if you now think about what limits, let's say, the Calvin Cycle in photosynthesis. And it's actually the CO2 fixing enzyme called Rubisco. Many of you know it's a BioG 101, and you could think it's actually a good catalyst. Yes, it is, but it's actually a very slow catalyst. So it takes only 5 to 10 CO2 molecules per second. At the same time, it also makes a lot of mistakes. It mixes up oxygen and CO2, and it's pretty hard to further engineer the enzyme. So you can make it faster, but then it will become less specific. And you can, at the same time, vice versa, you can make it more specific, but then it will become even slower. So it's like as human beings, it cannot be accurate and fast at the same time. So it's called the Pareto Optimum. And nature never broke the Pareto Optimum. So Rubisco sits exactly at a point. If you touch any of the parameters, the other one becomes worse. Now, this is what nature has evolved. Our thinking was, let's take another CO2 fixing enzyme. Let's take ECRs. It's a different class of CO2 fixing enzymes we discovered in bacteria 10 years ago. And those enzymes are 10 times faster than Rubisco. This is great, but they've never been explored for CO2 fixation in nature, for autotrophic CO2 fixation. And around this new class of highly efficient CO2 fixing enzymes, we then started to evolve complete new pathways. And this has basically allowed us to come up with a couple of different designs, a lot of theoretical designs. And one of those designs was the catch cycle, which we later on then realized in the lab. So we sketched out basically the topology, the individual metabolites. And after we evaluated its efficiency, we then went ahead and tried to identify the individual enzymes required to build the cycle. This has taken us probably screening and testing of up to 100 enzymes over, let's say, three to four years. And at the end of the day, we ended up with 15 of these enzymes that we could use to build the first version of the cycle. And then we had a couple of rounds of improvement, basically replaying evolution or mimicking evolution to make the system more robust and evolve into better efficiencies. Awesome. Yeah, that's super exciting. Are there any other major research thrusts that you're interested in at the moment? In principle, yes. So we have everything is basically, let's say, centered on CO2 fixation. If I look from the outside onto our lab, it's three research themes. One would be trying to discover new pathways in the biological dark matter, so new enzymes, new pathways that have not been described yet. The second part would be trying to understand how catalysis, in particular, CO2 fixation happens on a molecular scale. And the third would be trying to combine this understanding of catalysis pathways to develop new to nature pathways like the catch cycle and bring this back into artificial or natural cells and eventually long run into plants and then letting things evolve and learn the lessons from these evolution experiments. But it's a really interdisciplinary effort. It's really this basic fundamental understanding of the biodiversity, of the catalytic diversity, of active sites, and then the engineering part and the applied part at the end. So it's like this Max Planck quote, inside must precede application. So I really think understanding how pathways are made, how they evolve over time, what catalysis means allows you to build new options. And it's an interplay between trying, failing, learning from nature and going in circles. This is probably what describes our research best. So on that note, what are some of the biggest roadblocks in synthetic pathway and enzyme engineering? That's a good question. So different challenges. I think first and foremost, it's still our capabilities of deciding things on the paper so that they work in reality. As I told you, we designed the catch cycle, we picked the best enzymes, and still the system was far from being perfect. So we had to optimize in several rounds. It's like you write your computer program, and you think really you solved everything, and then you discover a lot of bugs in the system. So you go a second round, a third round, and you try to replace enzymes, you try to engineer enzymes better, you try to make the system robust. And this is something you cannot plan ahead, because also, if you bring enzymes from different backgrounds together, they sometimes steal each other metabolites, they interfere with each other, they don't have any coevolution, the parts are not synchronized, not optimized, not coevolved. And this is something we cannot easily predict. And then if you think about engineering enzymes, it's probably our incapabilities of predicting structure functions. So computational tools of developing a complete new enzyme we would need to fill a gap in our cycles. I think AlphaFold helps. I think the research done by David Baker and other people in the field who aim at engineering proteins is also great, but still we are not there yet that we can really design an enzyme denoble. This is still far from being solved. So this is computational tools, is our predictions, which we are still limited at. And on the other hand, it is probably also limitations in terms of screening technologies, right? So if you engineer enzymes, new variants, and we try to prototype new path, it's very much also building the infrastructure and the workflows of lab automation, maybe machine learning, again, like the cycle of innovation, build, test, learn where we have limitations. I think tools are out there, but I think it takes us another five to 10 years until we are there. We should be where engineering, for instance, is right already. So if you talk to an engineer in car industry, or if you talk to an engineer, software engineer, they're much, much better in predicting systems and simulating systems. I think we still have to learn a lot. And we have to accept that biology is a messy business to deal with. There's many engineering being, you know, you take these examples, the metaphors, the pictures from engineering parts you put together. Yes, that's true. But biology has one fundamental difference to classical hardcore engineering science. Biological parts are made to make mistakes because mistakes allow innovation. So every answer will make a mistake, because if it wouldn't do a mistake, it would be too slow, and or it wouldn't be flexible enough to evolve a different direction. So engineering biology does not mean to build the perfect parts. It means to build a robust and perfect system. And we still have to learn how to build this system. And it will be different from this classical hardcore hardwiring engineering. I think playing around and understanding how to keep evolution in shape, and this complex system shape, it's a different challenge we have to face. And I still think we missed some theory in this respect. So it seems like there are two thrusts here of ways that you could go about identifying enzymes. One of them is this de novo design approach. And then a second, what is a larger scale screening effort that might also involve some computational aspects to it? Which one of these are you most excited about? In an ideal world, which one would you rather be doing? I think the beauty lies in a combination of both worlds. If you look into recent enzyme engineering papers. So typically, you need to establish an activity from zero. And once you are there, you can actually throw experimental evolution at it, right? I think that's the power of biology, that you can build systems where you can optimize a system, you can just harness DNA encoded blueprints, right? It's a self optimizing system with good screening capabilities or selection strains. I think the future will be exactly what you said. We will have an initial part of design, computational design in the beginning to establish an individual activity, and then we harness actually the power of experimental evolution to move forward. And we don't see competing approaches. I would actually say you take the best of both worlds and you use whatever you get at your hand to move forward. And again, if you can let it run in the lab and self optimize it, it's much better than sitting there and letting everything compute. The problem also is that from a fundamental point of view, let's say optimizing a protein-protein interaction or protein binding interaction, that's simple. But trying to mimic a transition state where you have bonds half broken, this is pretty hard to predict. So this is also where limitations still are. And I think their evolution is much better in kind of moving on and trying to optimize a given system, time and space, because many of the mutations are not at the active site. There will be on the outside of the molecule, there's also protein dynamics, breathing, which you can hardly predict. Again, I think a combination will be the future of our field. Yeah, that makes a lot of sense. And so much of your work has involved in vitro cell-free models to create novel pathways. Are you looking to keep them in vitro or are you planning on the future to move them to living organisms, something like bacteria or even multi-celled organisms like plants? Right. Good question. I think we would like to deal with both for different reasons. So I could see that reconstructing biological systems, complex systems from the bottom up, it's per se a fundamental, interesting question. Can you build a system that can coordinate 50 different reactions in time and space? That's something that chemistry cannot do if you think about chemistry, right? So in chemistry, you have one, two reactions, you purify your product, you run the next two reactions, you purify your product. It's really complicated. It's a linear thing, right? Biology doesn't need to be linear. You can run 50. We have demonstrated 50 reactions in one pot. At the same time, you get a specific product from CO2 all the way to terpenes, right? And we've demonstrated this. So we can do that already. And if you have the same principle, throw back to chemistry, just think how chemistry and synthetic chemistry would look like in the future. So this is why I think the bottom up design, let's say artificial networks, minimal cells, reaction networks is a fantastic opportunity to learn still a lot about how to coordinate complex chemistry. On the other hand, of course, if you have a system that can self propagate and self evolve, this is where it becomes interesting in terms of the biology. And I think the fundamental challenge here would be to take a complex system, 17, 20, 30, 40, 50 different reactions you optimize, and you then transplant into chassis. I think again, there's a fundamental chance versus biologist, how does this new to nature network interact with the already existing genetic and metabolic network of the cells, our cells, modern cells plastic enough to adopt these new pathways? Where are the limitations? How much reprogramming do you have to do? It's almost like you have a hardware, like a computer, you try to play new software on what a modern cell accept this or do you need to go back to a stage with a minimal cell, and then you can evolve it. So for me, this is totally unclear. And I think this is interesting, challenging, but eventually also very much rewarding if you can rewire this metabolic system of cells completely. And of course, we try to do that. But you also try to be realistic. I think the first steps would be going into let's say, E. coli or small micro organisms. And we've done this the catch cycle, we are currently implementing parts of the catch cycle, partly it works already in inside of E. coli. And then we slowly forward and learning how the system adopts evolves over time, and taking this knowledge and trying to be a bit more, let's say predictive and having a bit more knowledge of how to implement into higher organisms. Challenge comes with that. Is of course technologies, how to read and write genomes. So how do you bring 17 enzymes in E. coli? I can tell you we've achieved already 14, 15, it's not too complicated, but how to bring 15 or 16 or 17 enzymes into a plant, and how to coordinate this across different organs. This is of course a technological challenge. And I think again, technology will drive our abilities, capabilities, with a catch we push pretty much. But again, like if you don't set yourself visionary goals, you won't never be there. And I think it will require both technology development, theory development, and we'll get there. That's a really exciting vision. And it seems like you have a lot of work to do over the next few years to be able to get there. At the same time, there are several companies that are working on different types of carbon fixation, not necessarily carbon dioxide. These include companies like Lansotec and New Light. What are some of your thoughts about commercialization opportunities in the short term and also in the long term for carbon fixation? And what on the horizon are you most excited about? So what I like very much is that biology becomes again the center of attention. So I think for a long time, we've ignored biology or life science in general as a potential solution to the problem of climate change. And what I really like is this combination, let's say, of chemical processes with a biological system. So let's see, for instance, Lansotec, where you have, let's say, CO, H2, CO2 mixtures that come out of chemical process, and then simply use living systems to convert this into useful products. You have other companies called ElectroArchia, for instance, and disclaimer, I don't have anything to do with it, but it's just a fascinating other example. Where you take electricity, you produce H2, CO2, and then you throw archaea on top of it and you make methane out of it. And I think, again, you can combine the highly efficient conversion of, let's say, renewable energy into reduced compounds, and then you take the power of biology to diversify the product scale. And if I think about short term, long term goals, so short term would be you try to engineer, metabolically engineer those organisms to produce a certain product, but you will still have, let's say, limitations how efficient the overall CO2 fixation rate is, the yields are. And so the long term goal would be to really establish, let's say, designer CO2 fixation pathways that get the maximum out of the conversion rates. So you have to spend less energy, which means more product you can make at the same time, you can increase space time yields. I think that is where I see the long term evolution of the field. First, metabolically engineering the organisms to, as a group of principle, get a certain product up and running, and then going in there with the synthetic biology knowledge and really engineer much more efficient pathways to improve yields in the future further. And I think that is where the field is, and I'm really excited to see that we are taking off slowly, but steadily, and we also need, let's say, tons of CO2 that can be fixed. So we hope we still need to scale it to gigatons. That's a challenge. Commercialization. As a fundamental scientist, I, of course, think a bit more about, let's say, future technologies as I sketched you, thinking about how to write and read genomes, how to design pathways, the workflows, what we learn, which chassis organisms are the best ones. Still, we do have a couple of short projects where we don't have, let's say, the catch cycle with 17 enzymes, but we talk about three, four, five enzymes that we already implement in microorganisms and or even plants. And we even have patents and or industrial partners at hand. We have this intermediate solution. We boost photosynthesis, let's say, or boost CO2 fixation, but not completely replace it. I think, again, this tells already how we think. Along this 10, 20 years, we need to develop a completely new pathway. There will be a first, a second, a third wave of getting into more complex designs and more complex applications in the long run. And I think it's actually nice, if you're a biologist, that you can think about stepwise milestones, two, three, four step enzymes, pathways, and then the bigger ones, which are more complicated to realize. That is super exciting. So maybe switching gears just a little bit. Throughout your career, you had mentioned that you've worked in Germany, Switzerland, and the US. What do you see as some of the biggest differences between the approaches of these countries to doing biology research and commercialization since we just talked about that too? Good point. Let's maybe first start with what is similar, right? So I think in all three countries you just mentioned, I've seen science be done at a really high level. So I think Germany, Switzerland, and the US are really the leading countries of the world where you have really cutting edge science being developed. So it's a really great environment. Now differences are, of course, critical size to some extent. Switzerland is, of course, the smallest of the countries, right? It's probably eight to 10 million people. Germany is around 18 million, and the US, you know, it's 300 or... Which means to do a certain kind of research type of research, you would like to have a critical mass of scientists, right? You need to have people that inspire you. You need to have a right environment. You'd be able to collaborate, cooperate, and of course need also a couple of labs competing with you in a friendly way, right? Because it's challenging to be really productive. So I think that is where there is a small difference. I would say Germany is the right mixture of being not too small and not too big. So it's pretty comfy. It's like a small town. The US is like a mega city, and Switzerland is like a really small village, and no offense. But you can already see how this leads to different, let's say, to different dynamics of the scientific system. What I also see is, I think that, let's say, in Switzerland and Germany, currently the funding situation is probably much better in terms of funding fundamental science. I think there's still a sense for that a certain type of science needs to be just science to understand fundamental principles. And there is a lot of basic funding still around, and you don't have to justify research with, let's say, application at the very end. So I think the US has become much more competitive. I'm also serving in different panels and or evaluating grants. I see that the competition is pretty, pretty high, and it's a bit over the top in certain cases, I feel. If you restrict science too much on the output, you get less creative at the end of the day. And you try to invest in something that's already rolling. And I think part of science is also failing, because from failing you learn. And so I hope that the situation changes a bit in the future in the US. I think Germany is doing a bit better here. So now if you ask me about the commercialization, and I would say what's really great in the US is that there's a much more entrepreneurial-like behavior. And people really think, as much as I criticize, think about application. I think many grad students, many posers I've met, have really great ideas, and they're not afraid of just taking on the challenge saying, let's try to do a start off. Let's try to just raise some money, go somewhere, and get an idea I have translated in reality. And I feel that the German system, the European system, is much more conservative here. So our graduate students typically go more into the big companies. Arguably, we have great companies in Europe, right? But I would see that they are much more afraid of taking their own fate in their own hands. And I think we can learn a lot from the US system. I also think that there's much more business money, general money around for investments. I think the investors are much more taking risks here. And this also drives the system in terms of innovation. So I like the system much more. I think we can learn a lot. Now Germany is more strategic here. So Germany has a very interesting system where you have, let's say, fundamental science that does Max Planck. It has another society, which is called the Helmholtz Society, which is half fundamental science, half industry collaborations, and then of course you have industry. So they try to push it in a very strategic way. I'm not sure if this allows to drive innovation completely. So new fields are probably better off. If you have a lot of start of spin off companies, some of them will survive and will become met mega players. Whereas in Germany, it's more strategic and step by step. And this leads also to some conservatism in terms of your new technologies. I admire the US in this respect, and I think we can learn a lot. And yeah, this is sometimes something I wish I could put here and import a little bit more from the US. This openness and this is really just go for your idea and just try to make it. And if you don't make it, that's fine. You can fail. And that's something also the failing culture in the US, I would say is much bigger. You can't be proud of having tried for spin off three died. One was a success. That's great. Right. In Germany, it's much for once you fail, you have this stamp your forehead you failed. I mean, how many of your experiments in your life fail, right? I mean, in my as I was a PhD, 90%, right? And you just get the next shot and it works. So I think this is also something we have to learn the failing culture is something which is much better in the US than in Europe in general, I would say. So earlier, when you were talking about these different research ecosystems, right in the United States, in Germany and Switzerland, and thinking about them as distinct units, but ultimately, they're all part of sort of a larger research ecosystem that that spans the globe, right? What do you think about the potential for different types of collaborations between different countries and ways that we could encourage those to happen in a healthy way? It's a good point. So I think, first of all, science is international. I think goes beyond boundaries. It's and what I really like about the scientific community is that all scientists I've ever met, he or she whoever speaks to me, is really excited about doing the best to to advance science. And so I think we actually ambassadors for a better society for better for a better planet in the future. And I think this excites me a lot. Of course, there's differences in a way where the country can can finance or fund, I wish sometimes we had more, let's say, transatlantic or international collaboration, funding opportunities, there's rarely things like the Volkswagen Foundation grants, we can work with an American partner, or you would have the HFSP human frontiers, I could see that we need some more international grant system, because you know, if you are cutting edge, you probably won't have everything in one country, even the US is big, I could see that some some great labs are sitting somewhere else. And vice versa, even though Germany also has a really great driving community, a couple of things are maybe better off in the US. If I look a bit more in my field, I could see that, for instance, biocatalysis, maybe also bottom up biology, that to some extent, this artificial cell is a bit more developed in the in Germany, where I think the synthetic biology community programming organisms, right, and some value on an organismic level, it's much more developed in the US, I think somewhere in between one can come together. I also think that certain computational approaches, large scale approaches are maybe better off in the US. Whereas Germany is a bit more on the fundamental side of small smaller molecular frameworks. And again, I think if the two worlds come together, you can learn from each other, you can you can basically help each other. I think it would be also my my vision of bringing bring the countries and different approaches together. Because I also think honestly, and you realize that once you go abroad, how much of a of a certain person you are to go up in a certain system, I never I never experienced to be so much German when I when I came to the US or to Switzerland, you know, I thought, well, whatever, I'm German at all. And suddenly, I know I miss my bread, the beers different way, really, really organized, right, it has a clear schedule, a clear things in all the things I never thought about me being a German. Turned out, I'm real German, but only to learn that when I was in the US. Of course, I picked up a couple of things from the US, I hopefully transport back and try to make the best of both worlds. But I see there's also a cultural difference, how you how you approach science. And I'm not saying it's good or bad. I think it's in the multilateral world in a in a diverse world, you try to appreciate the differences and try to learn the best approaches and use the best approach to follow for you for the for the forgiven challenge. I think this is where we can really succeed as a scientific community. It was very philosophical. I'm sorry. I don't know if I got it across. No, no. Yeah, I think that's been great. And another question that we have talking about these different types of scientific collaborations and moving forward in your career. Do you have any advice for students or postdocs right now who are looking to become professors? Jesus, don't become don't plan to become a professor. I think what I always try to be is a good scientist, right. And I always try to pick really cool projects, very interesting questions, things that really excited me. And I think all of us think about a PhD, right, you have to invest times of your life, right. And to be honest, to be frank, you have to work like, like, like crap, you have really to be in the lab, right? You're underpaid, right? There's a lot of things going wrong. The private life suffers, right? Oh, man, this long time experiment and you cannot just go for vacation. So it sucks, right. But then again, you have a great privilege because you can redo what you want to do, right. And if you find the right project, if you run the right scientific question, you find the right environment, the right PI, right, the right lab, it can be really the best time of your life. And I think I always try to do that. I always try to find a question, a lab, and try to be as good as possible. And, and I move forward, right. And again, what I said, you also have to accept that certain things, if you believe in your idea, it might not work out, but it has been worthwhile to try when I, when I sketched the catch cycle in the beginning, I knew exactly it would be a lot of challenges, right? A lot of anaerobic enzymes, certain steps. We didn't have an enzyme yet. And I was just hoping that the grad students or the PhD candidates would pick up the challenge and develop it. And I had to learn to let go. I've learned to accept that certain experiments, certain risky projects won't work, but it's worthwhile to try. It's like Columbus, right? You just have this crazy idea out there. There's a new continent, actually not a new continent out there. There is India, and you just take the other route compared to all the others, and you discover the US or America. Great, right? But you actually have to take the guts to take a small nut, what is it, a nutshell, really small ship, and just open ocean, you go somewhere. And if you're lucky, you will become the next Columbus or become a professor. That's it. I'm sorry to say so. Of course, it also on the environment you have to just to be honest and pay credit to the grad students, the postdocs, you know, me sitting here, when I was on my way to get tenured, I was not standing in lab anymore, right? It has been the great people, it has been my lab, it has been all the people really developing and trying to push hard that made it possible. Without my lab, I would have not had these publications, which at the end of paid off, right? So I think it's really also the people you work with, which make a difference. And this is, I think, it's what you also have to accept. It's at the end of the day, not only in your hands, it's all the hands, very much in the hands of your co workers. So what do you do to create an environment where the people that work for you can become the best scientists that they can be? That's pretty hard. We are now a team of 45 people. That is big, right? So you can imagine how do you create a healthy environment where there's no competition, right? I think, first of all, you agree on having no competition, right? And you agree to have a common vision. You agree that this big vision comes with a lot of risks, but it's also worthwhile to take on the risk. And it's worthwhile to set yourselves milestones, let's say also certain steps along the way. You know, if I had defined the catch cycle of we want to have artificial CO2 fixation in the plant, no way. But now breaking down in small, let's say small steps, small milestones you can reach, I think that makes total sense to me. It also motivates people to kind of be part of a huge enterprise. And I think that is important. It's very much important to form also a team that's healthy, that you bring in different expertises, different cultural backgrounds, you appreciate the culture of differences. You talk about that also, you talk about also failing, you talk about expectations, you manage people in a way, right? It's HR, it's motivation, it's a lot of individual meetings, you also have to accept that in big team, you don't have the direct supervision you would have in a small team, you need also to find the right people to take on this challenge. Then you create, let's say, a team that supports each other, and you get different ways of communication, let's say, whole team communication, lab meetings, shorter subgroup meetings, then the teams themselves develop their own, let's say, brainstorming sessions. And I'm just watching from the outside, I'm trying to navigate through, I'm still learning, right? I'm still young. I came from three PhD students to a group of 45. And this is pretty hard and pretty tough, especially if you have additional tasks like managing an institute, being active in different societies, evaluating a lot of other grants, keeping the business up and running. So I think we're doing well. I always think I could do better. I also have a private life, right? So there's also, I have to sacrifice on that side, the corona pandemic hasn't helped a lot. I think it's expectation management, it's honesty. And it's also, to some extent, accepting that you cannot perform always best, but you try to give your best. And I think I've just managed to find a great team of people, and we work together. And I think we also are openly communicating what is going well, what's not going well. And I think this helps a lot. And for sure, this is something we have to work on all the time. Yeah, it's great to hear your perspective on that. And that is excellent advice. So another question that we like to ask our guests a little more of a fun one, in an alternate reality where you didn't become a professor, is there anything else you could see yourself having done? I see a typewriter in your background there. Oh, wow. I was close to study literature. So you know, I think the typewriter is actually good. I think also you have to be really good in communicating results in an easy digestible fashion. So I think part of my profession is being creative and also being great in writing and or designing your slides. I think I have this kind of element of an eye for the arts, I mean, for the beauty of arts. It's something I really like to do. I also like to be an outdoor person. So if I was not just a crappy runner or cross country skier, I would have been better. I would have now been Beijing and compete for the Olympics. No, no, seriously, I mean, I think I'm a multi interested person. Many of you are probably that too. And it's interesting to see if I interview, let's say, I mean, postdocs are a bit more already dedicated, devoted and have already a career path in their mind. If you go in for very early career scientists like PhD candidates, it's very exciting to see how open they still are in terms of which project you would like to work on right here or she. And or then also really open towards either going to industry, academia, it's still like in like in the blank page. And I like this flexibility. And I also realized the older you get, the more complicated it gets. And I still try to bring this with me and be open for new technologies. Machine learning, for instance, I've never done machine learning. Now we do in our lab, lab automation, never had this. Now we do in our lab. So now kind of also appreciate the input that comes with this new generation of people to your lab and trying to be still young at heart and not to be too conservative in the way we should do science. I think this is the end of any science. So if you ask me seriously, where I would be also good at, this is maybe not writing or drawing slides or painting or whatever. And I think honestly, I could consider myself running a startup business. If you take together what I'm doing every day now, it's more or less like forming a team we discussed about the challenges, have a common vision, setting milestones, managing a budget and motivating a lot of the people I'm working with. We're also having a lot of meetings, trying to solve challenges, trying to put also different teams together to collaborate across each other, versus microfluidics people, that's enzyme engineers with physicists. So this is the environment I want to be in and I really like to foster this interaction. So more like a facilitator, not managing, but helping to get this up and running like a catalyst, like good catalyst. Also trying to invent technologies and also convince funders and investors because science is a business. Max Planck has, of course, a great basic funding. I don't need to write a lot of grants. I still get paid for my brain. This is up front trust for a long time. That's Max Planck. But I still do write the grants and I still have to convince also, let's say, our scientific advisory board, for instance, that my science is a great science. So I think that's also part of the business. And this is what you also need industry. You manage a team with a budget, you have to convince the upper management. So work with people. I think it's pretty similar. And so this is also why I don't think that people that leave academia are actually an exception. I think they are well trained in labs. They have a lot of management skills, project management, soft skills, time management, supervision, and they can directly use it in industry. And so I see them actually being very successful in the setup too. And I think actually people staying in academia are the outliers. Percent, right? And it's not a failure, actually evolution of a human being, of becoming a great person that works in industry. Well, that's a really beautiful vision of career progression. And it's great to hear about all of these skills and how they're translatable to different industries. As a final question, I wanted to ask if there's anything that you'd like to promote. This could be things like research openings, papers, books, or if you just have any wisdom you'd like to share with the EBRC community or anything that you'd like to share that you haven't yet talked about in the interview. Wow. So yeah, my wish is my two cents. I don't know if there's two cents. So, no, I honestly think science for me is on the one hand understanding how the world works, right? And then we talked about this. At the same time, it's also providing solutions to the great challenges of man. We are moving into the big challenge of climate change and science has to offer solutions. I think we need to work towards this goal that we can provide these solutions. I think we should pick ambitious goals. I think we should really try hard to develop biology as one of the solutions. And this means on the one hand, developing technologies, genome writing, how to build new to nature systems, enzymes, pathways, implement them. And we need also to convince policymakers, stakeholders that some of these technologies need to be implemented one time in the near future. And I think we have not the luxury to choose from, you know, at some point, the need is really high to implement certain technologies. And I've learned there's a sweet spot when people are not risk averse anymore, where people accept that new technologies will help and will provide a better world of tomorrow. I think we need to convince people. I think we need to communicate much more. What you're doing, guys, I think is really great because it helps us also to reach out to the public. And I think science and scientific decision making based on facts, it's in these times, really more important than ever. And I really hope we can push this forward, our agenda forward. We listen, of course, to what the concerns are. But you know, every technology comes with a risk, but also benefit. And you know, maybe GMOs are a solution. Maybe our plants are a solution, maybe just containing them in a fermenter is a solution. But we should not just clear out ideas because they are not currently accepted technologies. You know, mankind always has evolved. And I think there's a great potential in biology. If you think about how we have used computer sciences, right in the past, open science, open community, we have open databases for enzymes, we can actually make enzyme engineering maybe in the future, a task for high school kids that sit there and just drag and draw a new enzyme active sites or in the future might order even at some point DNA and screen, you know, a lot of things there. But we need to convince the public and the society that this is the way forward. And I really hope to do that. I really hope that you can achieve that with your with your podcast. I think we as a global community can work towards the goal and are not afraid of picking these challenges. And you know, if my catch cycle is solved, we'll solve it or not. I don't care if anyone can solve it with any sin bio solution. And I think that is what excites me most, trying to push science as one of the technologies that helps to improve life on this planet. Oh, man, this was too visionary and too philosophical. Again, I'm very sorry. We also need to give a vision right to in a world where we all get this negative news, right. And I think to some extent, inspiring and trying to say, look, there's something we have to invest in it. We don't know if in 50 years, it's a technology, but it might worthwhile to look at. This is probably the is probably the way forward for us and accepting that we have to fail and accepting that the next generation will learn from our failures. Now, that is a great final message. I'm excited for the vision you outlined. So hopefully that comes true. Yeah, well, thanks so much for coming on the podcast, Toby. It was a pleasure speaking with you. Thank you so much. Of course, I cannot leave this podcast without saying if you're interested in our research, always write me an email. I'm excited about PhD students and our postdocs, very international environment, very different from what you've probably maybe experienced a bit crazy, but open for many great ideas and a lot of freedom in the lab. So whoever is interested, just write me, write our lab and email. And then let's see if you get the best people to Germany and the beer is really good here. Perfect. Thank you for having me guys. It was a pleasure. And I hope to meet all of you at some point at a conference. I'm really missing conferences, guys. I cannot imagine two or three years without any political conference, poster presentations, a beer at the bar, could it be coffee at the bar? I don't care. It doesn't need to be alcohol. But I think all these things have been really problematic. And I think many of you also struggle just being not sure about the next step in your lives and just not having the network I have had, you know, and I really hope we can meet each other this year. 2020 is our year. It's a year of science and we can meet each other in person. Hopefully. This is the year. Yeah, I hope so too. Yeah. Thanks again. This has been another episode of EBRC and Translation, a production of the Engineering Biology Research Consortium Student and Postdoc Association. For more information about EBRC, visit our website at EBRC.org. If you are a student or postdoc interested in getting involved with the EBRC Student and Postdoc Association, you can find our membership application linked in the episode description. A big thanks to the entire EBRC SPA podcast team, Catherine Brink, Fatima Enam, Andrew Hunt, Kevin Reed, Rosh Jones, Coke Zealy, and David Mai. Thanks also to EBRC for their support and to you, our listeners, for tuning in. We look forward to sharing our next episode with you soon.